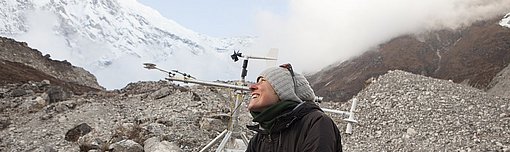
High Mountain Glaciers and Hydrology (HIMAL) ¶
Head: Dr. Francesca Pellicciotti
Contents ¶
Glacier shrinkage is one of the most striking signals of climate change and has profound impacts for downstream and coastal communities. High altitude glaciers, difficult to access and little understood to date, play a key role in contributing to streamflow in many of the poorer regions of the world. For these areas, where water is a vital supply for crops and communities, understanding changes in the high-altitude cryosphere is crucial to assessing vulnerability to future climate change.
We use a synthesis of observational methods to span scales of cryospheric and hydrological change, from local, process-oriented field research to catchment- and regional-scale remote sensing. We use observations to drive physically-based radiative, glacier and hydrological models for the recent past, present day, and into the future. Our research seeks to understand key processes in distinct study sites, and we leverage novel observations and understanding to produce robust ablation and streamflow projections for the Alps, Andes, and High-Mountain Asia. Understanding debris-covered glaciers and their role in the water cycle has been a key recent focus.
Research in pictures ¶
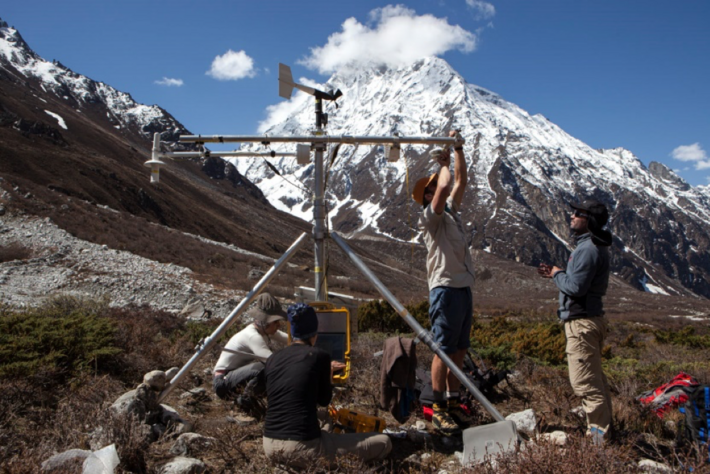
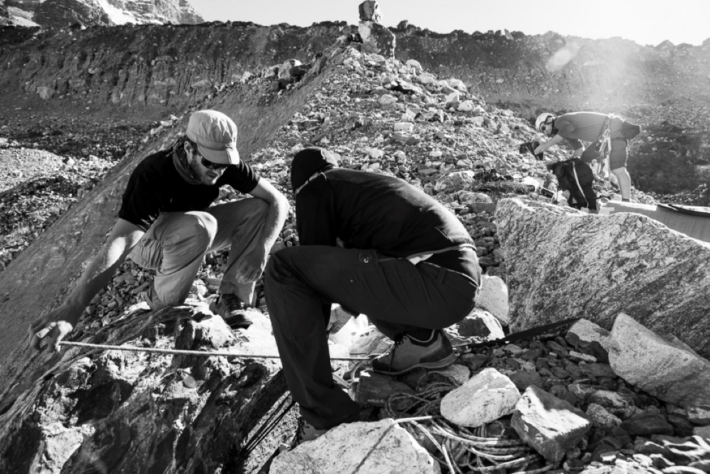
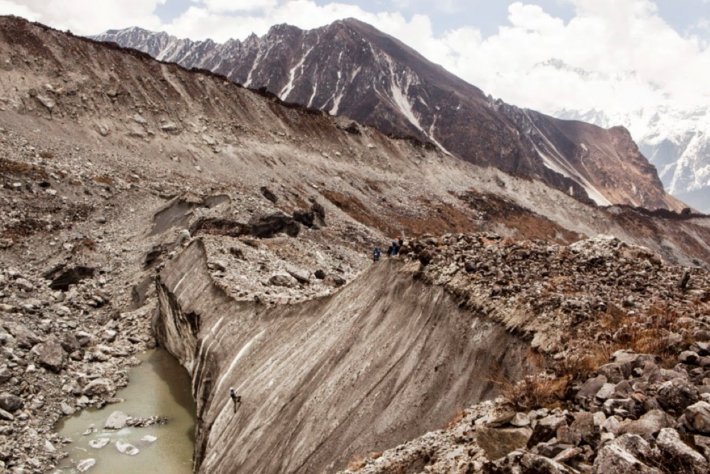
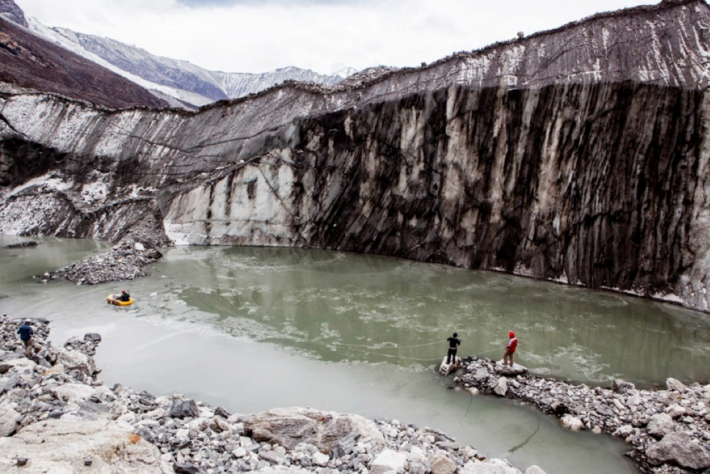
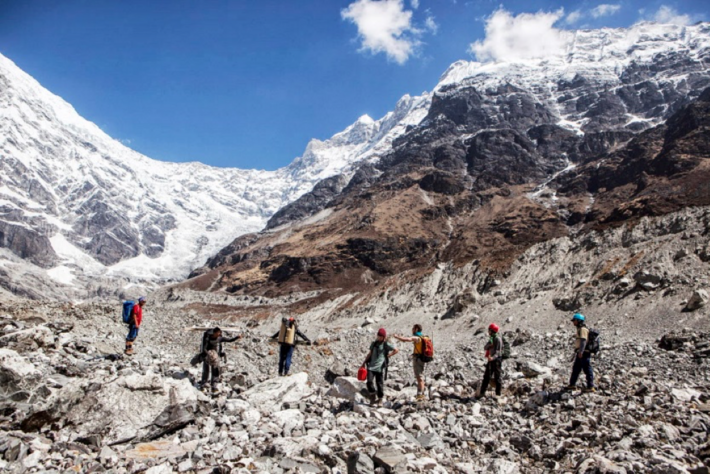
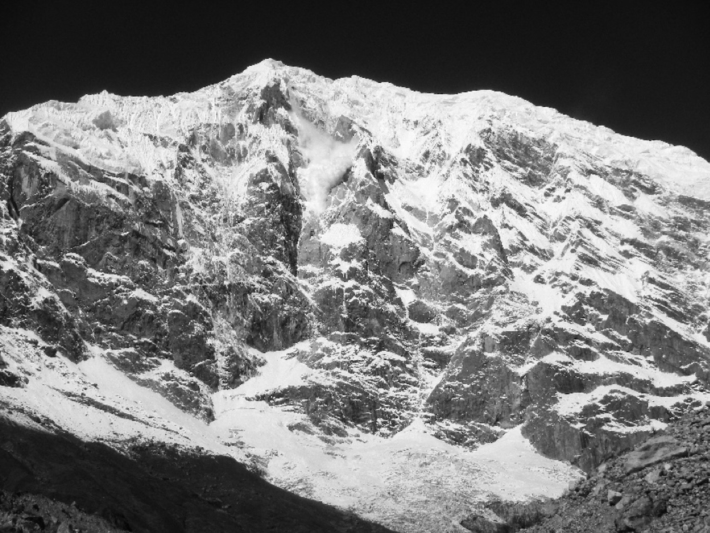
MSc topics ¶
Mapping supraglacial pond and ice cliff seasonality using PlanetScope data ¶
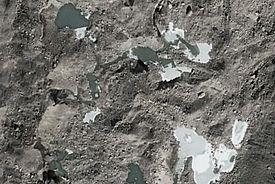
Supraglacial ponds have been identified as an important component of the hydrological cycle of Himalayan debris-covered glaciers (Miles et al, 2016, 2017; Benn et al, 2017; Irvine-Fynn et al, 2017), while together with ice cliffs these features are key contributors to mass loss in the ablation area of these glaciers (Thompson et al, 2016; Salerno et al, 2017; Brun et al 2018; Miles et al, 2018). Research has indicated possible seasonal patterns for both features (Watson et al, 2016; Miles et al, 2017; Steiner et al, 2019), but observation of these features during the ablation season has been limited by the consistent cloud cover and heavy rainfall of the South Asian Monsoon. The aim of this project is to use rapid-repeat multispectral imagery from Planet Labs to characterize the seasonality of supraglacial ponds and ice cliffs for selected debris-covered glaciers. You will use a novel mapping approach developed within our group (Kneib et al, 2021) to identify cliffs and ponds at high spatial resolution at a monthly time step since 2016, then compare the seasonal patterns and spatial coverage of ice cliffs to enable improved melt and hydrological parameterizations.
Re-evaluating gradients of temperature and precipitation for hydrological modelling in a glacierized catchment of the Nepalese Himalayas ¶
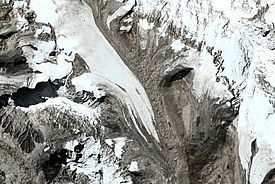
Realistic meteorological forcing fields are of utmost importance for reducing uncertainty in hydrological modelling. This is especially true in areas with complex, high-elevation topography, where meteorological variability is high, and where the impacts of climate change are most visible. The Langtang catchment in Nepal is a benchmark site for glacio-hydrological modelling in the Himalayas and monitoring has existed in this catchment for more than two decades. Distributed glacio-hydrological models (e.g. Immerzeel et al., 2012; Ragettli et al., 2015), have been driven using vertical and horizontal gradients of precipitation and temperature taken from literature or determined from the existing station network (Immerzeel et al., 2014, Heynen et al., 2016). More recently, efforts of data collection in the area have been expanded, new instruments have been added, old ones were renewed or maintained (Steiner et al., 2021). At the same time, new satellite products, improved climate reanalysis products (e.g. Hersbach et al., 2020) and new developments in climate-model downscaling and high-resolution atmospheric modelling (Collier et al., 2015) have been introduced, providing a wealth of information on the spatial distribution of meteorological variables which is yet to be explored. Given these developments and the input-requirements of modern, high-resolution hydrological models, a new blueprint for evaluating meteorological gradients in high-elevation catchments, based on multiple and diverse data-sources, is needed. This master project will re-evaluate the gradients of temperature and precipitation in the Langtang catchment, leveraging the extended and expanded in-situ records, combining them with reanalysis products and high resolution climate model outputs and comparing them with standard environmental gradients. The sensitivity of a state-of-the-art land surface/hydrological model to these new values will be tested.
Processes of debris cover change on small alpine glaciers ¶
Haut Glacier d’Arolla has been a test bed of glaciology research, especially since the early 1990s, when pioneering studies of its meteorology (Strasser et al., 2004), melt (Pellicciotti et al., 2005), hydrology (Willis et al., 2002; Nienow et al., 1998; Arnold et al., 1998), and glacier dynamics (e.g. Mair et al., 2003) significantly contributed to the development of glaciology. One of the earliest studies of the formation of medial moraines (Small et al., 1979) also included Haut Glacier d’Arolla as a study site, although at that time the debris was limited to narrow medial and lateral moraines (Figure 1). However, like many alpine glaciers, Arolla has receded in recent decades, although this has been accompanied by a gradual increase in its debris cover. Despite large recent advances in the mapping of supraglacial debris cover and its change (Stewart et al., 2021), and in sub-debris melt modelling (see Reid et al. (2012) also on Arolla), the understanding of the processes causing debris to increase on retreating glaciers is limited, being confined to mainly modelling studies (e.g. Anderson, 2000) or very few field based studies (Kirkbride and Deline, 2013). This project will use a combination of traditional techniques (repeating the debris thickness measurements conducted in 2010 by Reid et al. (2012), measurements of englacial debris concentration and glacier velocity) and modern methods (repeat collection and analysis of UAV and time-lapse camera data) to provide a full picture of the rates of debris cover change and the driving processes which lead to debris cover formation on small, retreating alpine glaciers. Research questions to tackle could include: what factors result in the growth of medial moraines? What are the conditions required for debris to accumulate on the ice surface? Which mass movement processes result in debris movement and the growth of debris covers?
Figure 1. Haut Glacier d’Arolla as depicted in Small et al. (1979) (left top panel) and in Reid et al. (2012) (right top panel). Lower panel showed debris thickness change over the glacier from 2002-2019 (Stewart et al., 2021).
Physics-constrained machine learning for glacier melt modelling ¶
Physically based glacier mass balance models require a number of physical properties (parameters) as inputs. Unappropriate assumptions or values from the literature often lead to poorly performing models and large biases in modelling glacier melt and freshwater discharges from the alpine cryosphere. These properties include e.g. roughness length of the glacier surface (Miles et al., 2017) or the thermal conductivity through a supraglacial debris layer (Brock et al., 2010). They are often difficult to quantify where direct observations don’t exist, i.e. almost everywhere. You will explore the synthesis of data-driven and physical methods (Reichstein et al., 2019) by testing different machine learning algorithms and architectures (Neural Networks, LSTMs,...) in combination with a state-of-the-art, physically based land surface model (Fatichi et al., 2012). You will use this hybrid modelling approach to learn key parameters for modelling debris-covered glaciers in a physically constrained way (Zhao et al., 2019). This is a promising avenue to gain physically realistic estimates of glacier properties in a distributed way, while leveraging the vast information content of large datasets. Your datasets will include meteorological reanalysis (e.g. Hersbach et al., 2020), remote sensing (e.g. Landsat & Sentinel), high-resolution atmospheric modelling outputs (Collier et al., 2015), and in-situ station data from different locations in High Mountain Asia. Ideally, you have some basic experience in machine learning and you are keen to explore a very recent and emerging field of research.
Mapping englacial conduits in the subsurface of debris-covered glaciers ¶
Supraglacial cliffs and ponds contribute disproportionately to the melt of debris-covered glaciers, acting as energy windows at the surface of glaciers otherwise insulated from a relatively thick layer of rock debris. The formation and evolution of these features is highly correlated with observations of englacial conduits (Miles et al., 2017) and streams existing within the debris and at the ice-debris interface (henceforth sub-debris streams). Surface lowering in the ablation area can cause the conduit roof to sag and collapse, resulting in the formation of a depression surrounded by steep ice slopes, and sub-debris streams also affect glacier mass balance by promoting heterogeneous melting. Englacial conduits and sub-debris streams are thus a key component of the debris-covered glacier system and their systematic identification and characterization would be a major step in the understanding of these glaciers and their evolution (Benn et al., 2017). Direct observations of englacial conduits are limited to a few sites and difficult to repeat in time (Kamintzis et al., 2018). Another approach has been to use fine-resolution Digital Elevation Models derived from Unmanned Aerial Vehicle (UAV) surveys to map the subsidence patterns that are a sign of deformation of shallow subsurface conduits (Immerzeel et al., 2014). This project aims to apply this method to other available UAV and high resolution remote sensing datasets to attempt to map the englacial conduits and sub-debris streams. Then, the project will tie these observations to surface observations, both visually from the orthoimages and applying GIS tools for flowpath delineation from the glacier topography. The project will thus link englacial and supraglacial hydrology for debris-covered glaciers.
Tracking supraglacial debris motion using time-lapse cameras ¶
The melt of debris-covered glaciers is highly heterogeneous due to the high variability in debris distribution (Westoby et al., 2020). Cliffs and ponds act as melt hotspots as they are directly exposed to incoming radiation (Buri et al., 2016; Miles et al., 2016), and a thin layer of debris also enhances melt (Fyffe et al., 2020), while debris thicker than a few centimeters has a melt reduction effect (Ostrem 1959, Nicholson et al., 2018). As a result, the surface mass balance and topography of a debris-covered glacier is controlled by two competing drivers: Differential melt enhances the heterogeneity and the topography of the surface, which is compensated by debris redistribution (Sharp, 1949, Bartlett et al., 2021). This debris redistribution, which depends on slope, debris thickness, water content and properties, is therefore one of the main controls of the evolution of such glaciers (Moore, 2018). Recent studies have explored the stability of supraglacial debris and its evolution on debris-covered glaciers (Moore et al., 2019; Westoby et al., 2020) but the time-scale over which the debris is redistributed is still a large unknown due to the lack of high frequency and high spatial resolution observations. This project aims to address this question of how fast the debris moves on a glacier. You will use time-lapse camera time series of debris surface with hourly temporal resolution to derive surface velocity fields using cross-correlation techniques (Messerli and Grinsted, 2015). Glacier motion will need to be accounted for to be able to finally obtain accurate debris motion. The method will be tested for one glacier in Tibet, and expanded to other sites in different climatic and geomorphological settings (Nepal, Switzerland) if successful. This will enable to look at the climatic controls on debris motion, in addition to other controls such as the local slope and debris thickness.
Recession and thinning patterns of a calving debris-covered glacier in the Glarner Alpen ¶
With receding glacier tongues terminal lakes form and grow continuously in Switzerland (Mölg et al., 2021). They accelerate the mass loss at the glacier terminus via sub-aerial melt and calving, but also impact the glacier velocity and strain rates, leading to enhanced crevassing (Röhl et al., 2006). Receding glaciers can also develop a supraglacial debris layer originating from avalanching which reduces melt (Ostrem, 1959). For this topic you will be focusing on a glacier in the Glarner Alpen which is both debris-covered and calving, in addition to being highly avalanche-fed. Combining long-term remote sensing data and field observations (including debris thickness measurements and UAV photogrammetry) you will quantify the thinning and retreat of this glacier as well as the drivers of its changes.
Investigating responses of glaciers, streams, and vegetation to El Niño and La Niña conditions in Peru ¶
The El Niño Southern Oscillation (ENSO) has a profound influence on atmospheric conditions along the west coast of the Americas. In the tropical Andes, El Niño conditions are associated with strongly decreased precipitation totals, leading to considerably higher snowline elevations, whereas La Niña conditions are associated with increased precipitation (including snowfall) and maintain lower-elevation snowline elevations (Arnaud and Muller, 2001; Veettil et al, 2014). The stark differences between El Niño and La Niña years thus play a major role in controlling glacier mass accumulation and loss in the tropical climate of Peru, where seasonal differentiation is otherwise reduced to relative dry and wet seasons (Thompson, 2000). As glaciers can play a vital role in buffering streamflow during droughts (Kaser et al. 2010; Pritchard et al. 2019), they are likely to be crucial sources of water in El Niño years for downstream ecology and society alike. However, their role in this regard has not been assessed in detail. This project will investigate the contribution of glaciers and snowmelt to the river flow of a key tributary to the Rio Santa in the Cordillera Blanca, the Artesonraju catchment. The project will use the ecohydrological model Tethys-Chloris (Mastrotheodoros et al. 2020), and will consider glacier, streamflow, and vegetation responses for three periods representing 1) strong La Niña conditions (2010-2012), 2) moderate conditions (2012-2014), and 3) strong El Niño conditions (2014-2016). The project will investigate the differences in glacier mass balance modelled for the three years, the modelled discharge anomaly between the years, and differences in other key components of the hydrological budget (e.g. snowmelt, evapotranspiration and rainfall), feeding into efforts within our group to assess the Rio Santa’s future water security and the role of glaciers.
Examining the preferential orientation of ice cliffs around the world ¶
Ice cliffs have been pointed at by a number of numerical modeling and field studies as major contributors to the mass loss of debris-covered glaciers, due to the enhanced melt that takes place at their locations (e.g. Brun et al., 2018; Buri and Pellicciotti, 2018). The behaviour of these cliffs seems to be controlled by their predominant aspect, as on the few glaciers where they have been studied, there seems to be a high majority of north-facing cliffs. An explanation to this is that the incoming energy fluxes are more homogeneous on a north-facing slope, resulting in similar melt at the top and bottom of the cliff, while for a south-facing cliff the melt-gradient is stronger, leading to the top of the cliff melting faster, resulting in a decrease in the cliff’s slope and its reburial by debris (Buri and Pellicciotti, 2018). However, this has only been thoroughly studied in the Langtang catchment of Nepal, and there is little information on the decay of ice cliffs based on their orientation elsewhere in High Mountain Asia (HMA) or in other mountain ranges around the world. For this project, you will combine remote sensing and modeling to i) assess the ice cliffs’ preferential orientation for different glaciers in HMA, the Alps, the Andes and Alaska from high-resolution satellite imagery, ii) model ice cliff backwasting at these few locations with distinct settings using high-resolution DEMs derived from UAV and meteorological data from local on-glacier AWS. You will also participate in a 2-3 day field trip to Zmutt glacier to collect some more data on the ice cliffs there.
Evaluating methods for modelling snow albedo in glacierized catchments ¶
Albedo determines the energy uptake of the land surface through the partition of sunlight into absorbed and reflected radiation. It controls the amount and seasonality of meltwater from snow and glaciers, which are vital contributors to freshwater resources, especially in alpine regions. Snow albedo undergoes an evolution from refreshing to decay, shaped by a multitude of environmental factors and snow metamorphism, hence being a process difficult to capture with numerical models. Physically based hydrological and land surface models typically rely on parameterizations of varying complexity and calibration demand (e.g. Brock et al., 2000; Lehning et al., 2002; Klok and Oerlemans, 2004; Ding et al., 2017;), to mimic the albedo evolution related to snowfalls and snow cover. The goal of this study is to evaluate existing parameterizations against station data and field measurements at various sites in the High Mountains of Asia (HMA), using a state-of-the-art land surface model (Fatichi et al., 2012), and, if applicable, to find an improved parameterization of snow albedo. This way, one can also assess the effect of using different parameterizations on the mass balance of glaciers and the hydrological budget of HMA catchments.
Distributed air temperatures within boundary layers of small Alpine Glaciers ¶
Near surface air temperature (Ta) is a crucial variable for determining the surface melt of glaciers and ice caps, largely through its control on sensible heat fluxes. Moreover, for simpler, statistical models of melt estimation, Ta is often the principal (or only) variable considered (e.g. Hock et al., 2003; Pellicciotti et al., 2005; Petersen and Pellicciotti, 2011). Nevertheless, due to the lack of available data for high elevation, glacierised regions, the method of distributing Ta has often been a simplified space-time invariant lapse rate which ignores high elevation surface-air interactions and does not account for the cooling effect of the glacier boundary layer (e.g. Nolin et al., 2010; Kraaijenbrink et al., 2017). Accordingly, temperatures are often overestimated at high elevations and on-glaciers, resulting in large biases in melt models (Carturan et al., 2015; Shaw et al., 2017; Bravo et al., 2019). While some alternative methods have been recently developed to estimate Ta for glaciers (Greuell and Böhm, 1998; Shea and Moore, 2010; Petersen et al., 2013; Ayala et al., 2015), it is still unclear which processes determine the efficacy of those methods (Shaw et al.., 2021). Using existing and ongoing data collections, the aim of this project is to explore the local controls of Ta distribution in space and time to better understand how we might model its behaviour in statistical and physically-based melt models. The project will leverage both station and reanalysis data to determine the most suitable way to model Ta across several Alpine glaciers.